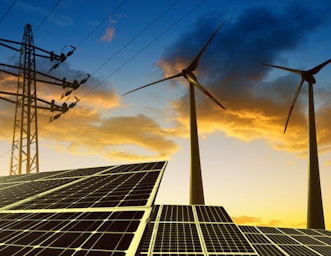
Photovoltaic (PV) Solar Panels
The price of Photovoltaic (PV) solar panels has dropped rapidly in the last ten years. A domestic PV array can now be cost effective without any subsidy. You can sell the electricity you don’t use directly for a fair export rate. Whether you use or export the power, PV is a great way of helping us get towards a zero carbon electricity grid.
It is possible to charge a large battery using PV solar panels. However, at present this may not be worthwhile in a grid-connected house. Batteries are still quite expensive, plus they have environmental impacts in manufacture and disposal.
If you want to use sunlight to heat water during the summer, solar water heating (solar thermal) is a more efficient option than PV. This means it would take up less roof space, although there may not currently be much difference in cost.
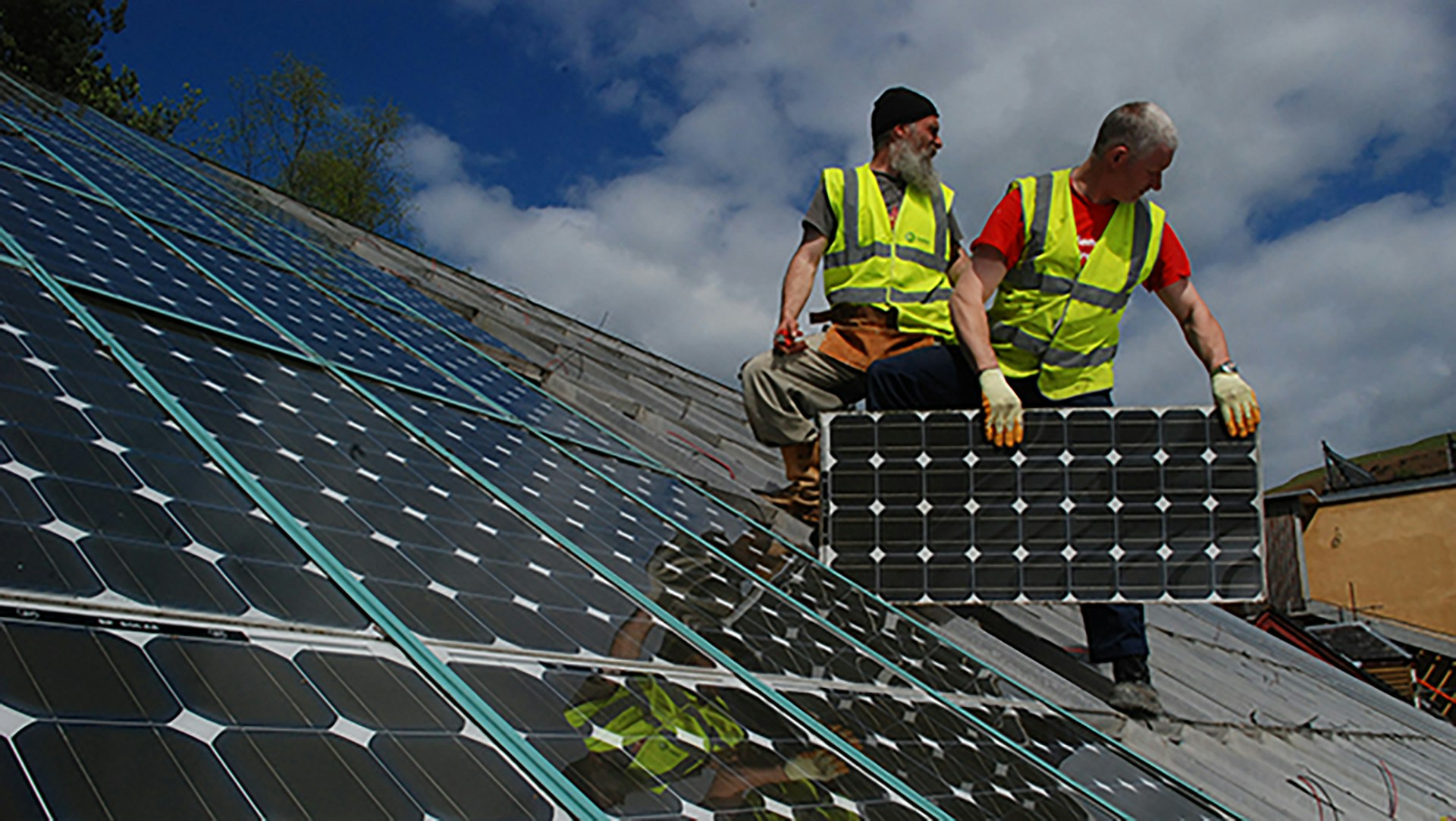
Is my home a good site?
A house roof is usually an excellent site, but solar panels can also be mounted at ground level. You need a site that’s largely free of shade, particularly between spring and autumn. Solar panels perform well if facing anywhere between south-east and south-west, at an angle of 20 to 50 degrees. A PV array that faces due east or west will give about 20% less energy than one facing due south. Roof mounted panels are usually a ‘permitted development’, so you won’t normally need planning permission.
How much electricity could I generate?
The ‘rated output’ or ‘rated capacity’ is a key figure to use when you compare PV systems. This is the peak power in kilowatts (kWp or just kW) that a PV array gives in bright summer sunshine. Domestic PV systems are commonly between 3 and 4 kilowatts, taking up 20 to 30 square metres of roof.
Of course it’s not sunny all the time, and the output of PV panels will drop a little under cloud or on winter days, when the sun is weaker. In the UK you can expect one kilowatt of panels to generate between 800 and 1000 units (kilowatt-hours, kWh) of electricity per year. So a well-sited domestic system of about 3.5kW peak output could produce around 3,000 to 3,500 kWh per year. Where you live will be a factor – for example Cornwall receives 30% more solar energy than northern Scotland.
For a detailed assessment of output by month you could use the European Commissions PV GIS online tool. Zoom in on the map there and click on your location, and set the correct slope and orientation (azimuth). The tool defaults to a 1kW array, so change the peak power to the sort of kilowatt rating you are thinking of installing.
How much does a solar PV array cost?
Prices collected through the MCS scheme (see below) showed that in 2021 prices dipped to about £1,500 per kilowatt for small installations. However, prices have since risen to about £2000 per kilowatt – presumably a combination of inflation and increased demand for PV.
That would mean that a domestic array of 3.5kW (about 25 square metres) might now cost about £7,000. Larger arrays of 10 to 50 kW (such as for a school roof) have a lower average cost – perhaps down to £1,000 per kilowatt.
Ongoing maintenance costs will be very low because there are no moving parts and solar panels should last for decades. The only major part that will require replacement every 10 years or so is the inverter, at a cost of perhaps £500 to £1,000. The inverter converts the low voltage DC output of the panels into the 230 volts needed in your home.
A domestic PV system will be particularly economic if you’re renovating a roof, or building a house from new. PV panels can be used in place of roof tiles, and many of the associated costs (such as scaffolding) will be incurred when roofing anyway.
What’s the payback and savings?
Getting about 3,500 kWh of electricity from solar panels instead of from a gas-fired power station will avoid about 1.4 tonnes of carbon dioxide emissions. Until all energy systems are decarbonised there will be some carbon emissions from the energy used in the manufacture of solar panels. However, the energy used during the manufacture of the PV panels is far less than they will generate through their lifetime. Even under UK levels of sunshine, a PV array will pay back this ’embodied energy’ in less than three years. After that, the panels deliver the full carbon saving per year estimated above. See the related questions below for more on this and the other environmental impacts from making solar panels.
For a new installation you can get payments for exported energy under the Smart Export Guarantee (SEG) scheme. To claim SEG payments you need a type of smart meter that’s able to measure exported electricity (which many first generation smart meters cannot do). You can sign up for SEG payments with a different company to your energy supplier, so do shop around for a good deal. Some offer 15 pence or more per kilowatt-hour (kWh) but some pay much less.
For a PV roof array producing about 3,500kWh per year, say you can use only about one quarter directly and you sell the rest to the grid. If what you use directly saves you about 22p per kWh (the July 2024 price cap on electricity equates to 22.36p) and you can export the rest for about 15p per kWh then the overall benefit would be almost £600 per year. That should mean a financial payback of between 10 and 15 years.
Electricity prices have gradually been dropping. However, even if we instead use an import price of 20p per kWh and export of 10p per kWh, the payback time may only increase to just over 15 years. Although that’s a longer term investment, it’s still well within the lifetime of the panels.
Most photovoltaic solar panels come with a guarantee that they will still be giving something like 90% of their maximum output after 25 years. So a PV roof is a long term investment that will become more and more beneficial over time. Payback times for energy saving measures may well be quicker, and so these should always be your first steps.
What type of PV solar panels should I use?
Most solar panels are made using either monocrystalline or polycrystalline silicon. From a practical perspective, there is very little difference between these two types. The output of crystalline silicon panels decreases very slowly over time. Some other types may be cheaper but degrade more quickly, so check the power output warranty.
Polycrystalline solar panels consist of visible crystals in different shades of blue. They are slightly less efficient than monocrystalline panels, which are dark blue or black with no visible crystals. This small efficiency difference just means that a 1kW polycrystalline array will be slightly larger than a 1kW monocrystalline array. Under identical conditions, both arrays will produce roughly the same amount of electricity and cost about the same amount.
How can I find a solar PV installer?
Finding a qualified professional installer who can offer you a good service at a competitive price will be important. As with any big investment it’s well worth finding a few installers to get quotes from to compare.
You can find installers by searching the listings of the Microgeneration Certification Scheme (MCS). All installers have to meet certain standards to be accredited, including signing up for a code of conduct such as the Renewable Energy Consumer Code. Their code is backed by the Trading Standards Institute and covers many issues, including ‘hard sell’ aggressive sales tactics. These schemes should have complaints procedures in place to follow up claims against companies that either mis-sell systems or do poor quality work.
Another option is a local group buying scheme, which should in theory reduce costs. You can check at Solar Together to see if your local council offers a scheme. If they do, you can compare their price to that quoted by installers.
Further information
For some additional details, see our questions and answers section below. You can also attend one of our courses.
Related Questions
How long do PV panels last?The life expectancy of a PV panel is likely to be 30 years or longer though there will likely be some cosmetic physical decay and a decrease in energy output.
Crystalline silicon PV panels should come with a ‘power output warranty’. This typically guarantees they’ll still be producing 85% to 90% of their initial rated peak output after about 25 years. This means the output is expected to decrease by about 0.5% per year.
Very few panels have been installed for long enough to need replacing because of diminished performance. In the UK, more panels were installed between 2006 and 2008 than in all previous years together. Only a small proportion of all PV panels installed globally are older than that.
Even early PV panels still good after 20 years:
The LEE-TISO testing centre for PV components at the University of Applied Sciences of Southern Switzerland installed Europe’s first grid-connected PV plant, a 10kW roof, in May 1982. When the panels were tested in 2002, the average peak output of the panels was only 11% lower than the nominal value in 1982. Between 1983 and 2002 the peak output had only degraded by around 0.5% per year.
Here at CAT we installed a large PV roof array back in 1997. After about 16 years we tested all of the panels and they were still mostly at 90% or more of their original rating. This was in line with the predicted drop in performance over time.
As with any industrial product there is an environmental impact associated with photovoltaic panels. The main areas of potential concern are:
- The energy required to produce them, and the fuel for this (see the question on energy payback)
- Toxic and other potentially harmful materials used or created during manufacture.
- What happens to them at the end of their lifetime.
It’s important to keep these issues in context. All electronic equipment leads to similar concerns, and whereas many electrical goods are only in use for a few years, most PV panels are expected to last for at least 30 years. Furthermore, PV panels are used to replace other sources of electricity that usually have a much greater environmental impact.
The main component of most PV modules is silicon. This isn’t intrinsically harmful, but the manufacturing process does involve toxic chemicals that need to be carefully controlled and regulated to prevent environmental damage.
Making monocrystalline panels tends to result in more waste, as they’re made from slices of silicon ingots – leaving offcuts. However, the waste can be used to make polycrystalline or multicrystalline PV modules, constructed of ‘mashed up’ silicon. Thin film silicon reduces the volume of material needed by spraying a thin layer of silicon on to a surface, so this has the potential to reduce waste and pollution.
A report by the Silicon Valley Toxics Coalition lists a number of potentially damaging chemicals used in the manufacture of PV cells. There are several different types of PV technology and each of them use different processes to manufacture, but they are some harmful chemicals commonly involved.
- Crystalline silicon is made using silane gas, the production of which results in waste silicon tetrachloride, which is toxic. It can be recycled into more silane gas but has the potential to cause harm.
- Sulphur Hexafluoride is used to clean the reactor used in silicon production. If it escaped it would be a very potent greenhouse gas. It can also react with silicon to create a range of other compounds.
- There are a range of other chemicals used for cleaning the silicon and cells.
Aside from silicon production, electrical connections & wiring can include lead and small amounts of aluminium and silver, although lead-free solder is available. The use of lead-based solder leads to pollution if landfilled or incinerated, so good collection and recycling schemes are important. It’s important to note that the same materials are in most other electronic goods, so we need to develop ways to control them and address potential problems anyway.
Some kinds of PV panel contain cadmium, which is an extremely toxic metal. However, when in the form of cadmium telluride (CdTe) it’s a stable non-metallic substance and is not soluble in water. The melting point of CdTe is 1050 degrees C, so accidental domestic fires would not pose a risk. Industrial fires may reach higher temperatures, but tests have shown that the molten CdTe remains contained in the PV module. It is also worth noting that one NiCd battery contains 2500 times as much cadmium as a thin film CdTe PV module, and the production of 1kWh of electricity in a coal fired power station will emit 360 times more cadmium (in air pollution) than is needed in each CdTe solar module per kWh produced. Cadmium is essentially a waste product, as it is only collected as a by-product of zinc mining and manufacture. So it will still need to be dealt with in some way, whether used in PV modules or not.
It will be many years before most PV panels come to the end of their life, so we do have time to make sure recycling schemes are in place and accessible. PV panels are covered by WEEE (waste electrical and electronic equipment) legislation, which governs the disposal of electronic equipment – making the manufacturer responsible for eventual disposal or recycling. Manufacturers and distributors of PV panels have come together in the PV CYCLE scheme.
A PV array operating under normal UK conditions will produce many times more energy over its lifetime than was required for its production. Some mistakenly think that PV panels don’t produce as much energy as they take to manufacture, but this stems from the very early days of the satellite industry, when weight and efficiency was far more important than cost.
Studies looking into this question typically conduct what’s called a life cycle assessment (LCA), often known as “cradle-to-grave analysis”, which looks into all the resources that go into the production, operation and disposal of a PV system. This includes the “embodied energy” used when mining the raw materials and producing the panels as well as the electricity they’ll produce. The energy aspect of the LCA is often expressed in terms of the time the system has to operate in order to produce as much amount of energy (or save as much carbon) as was required during production.
Studies draw different boundaries of what should be included in the analysis. For example should it just be the manufacturing of the panels, or also the structures the panels will be mounted on, or even a share of the total personal energy consumption of every labourer involved in the process. Assumptions about the operating conditions will produce different results – for example if the PV panels are installed in California or in northern Europe.
A study by researchers from the Netherlands and the USA (Fthenakis, Kim and Alsema, 2008) analysed PV production processes based on data from 2004-2006. They find that it took 250kWh of electricity to produce 1m2 of crystalline silicon PV panel. Under typical UK conditions, 1m2 of PV panel will produce around 100kWh electricity per year, so it would take around 2.5 years to “pay back” the energy cost of the panel. PV panels have an expected life of least 25 to 30 years, so even under UK conditions a PV panel will generate many times more energy than was needed to manufacture it.
Calculating carbon payback times introduces additional variables, especially the “carbon cost” of the electricity production replaced/avoided by the PV system: Carbon payback times are shorter in countries where electricity is primarily produced using coal power stations, and longer in countries where grid electricity is already produced by low-carbon technologies. But generally payback times for carbon are similar to those for energy.
A 2006 report by the UK Parliamentary Office for Science and Technology calculated a “carbon footprint” of less than 60 grams per kWh of electricity from PV in the UK (or around 35g per kWh in southern Europe), compared to 10 times as much for fossil fuels. Research by Fthenakis, Kim and Alsema, (2008) suggested that total greenhouse gas emissions for electricity from PV panels is between 20 and 80 grams of carbon dioxide equivalent (CO2e) per kWh (under UK conditions). This is far less than the emissions for electricity from fossil fuel power stations. From just burning the fuel (so not including extraction & delivery), gas-fired power stations will emit around 350 to 500 grams of CO2e per kWh, and for coal it can be as high as 1000g per kWh.
A number of companies produce solar tiles or slates, designed to have similar dimensions to flat slate tiles and so suitable for integration into this type of roofing. However, many of these do not have the visual appearance of actual slate. The tiles could still have a blueish crystalline surface, or be a matt or shiny black or grey.
As these solar tiles will still look different to slates or tiles, they may not be any easier to get planning permission for in situations such as a listed building, national park or conservation area. In those situations it’s necessary to check first with the planning authority. For most homes, any kind of solar roof where the panels either form the roof or are mounted just above it (as most are) will be a ‘permitted development’, so planning permission is not needed.
You might be able to find these solar products by going to the Microgeneration Scheme product listings and searching for solar PV products with the keyword ‘slate’ or ’tile’. Or you may just need to ask local installers to see what they can supply.
Integrated solar roofs
Bear in mind also that many types of solar panel can be fitted as an ‘integrated’ solar roof – with the panels flush to the tiles. If you need to reroof anyway, or are building a new home, putting in an integrated roof will save on tiling costs.
It is important to ensure that any integrated array – whether tiles or panels – has adequate ventilation to avoid overheating. However, even with these ventilation measures, an integrated roof is likely to have an lower output that an equivalent non-integrated roof.
Solar photovoltaic (PV) output will reduce a little when the modules reach high temperatures. As a rule of thumb, you can expect around 0.5% decrease in module output per degree centigrade temperature increase.
This does affect the design of roof arrays – as the modules need to be ventilated to prevent them getting excessively hot. An array that is mounted above an existing roof (a “non-integrated” array) will have a gap behind and at the sides, allowing air to circulate and heat to disperse.
Roof-integrated arrays, including PV slates or tiles but also other integrated systems, may not have this circulation. If they don’t, the array will be prone to getting hot – making it less efficient.
For a new house, this ventilation can be designed in.
For existing roofs there are measures that can help improve the performance of an integrated array. One option is ‘counter-battening’, which involves adding extra battens above the sarking but below the conventional battens (and at right angles to these), as in the image below. This would of course mean some extra roofing work, including removing all slates on that pitch – not just those being replaced with PV tiles.
Or companies might use a special fitting system designed to allow for cross-ventilation.
Even with extra ventilation, an integrated array will tend to have a slightly lower performance than a non-integrated array.
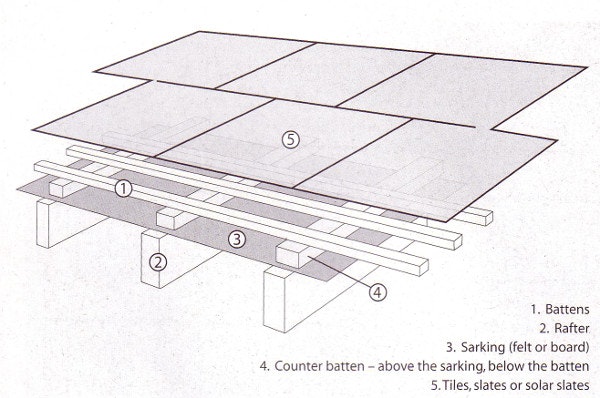
Roof mounted panels are usually a ‘permitted development’ as long as the panels are flush with the existing roof. This means that in most cases you won’t need to apply for planning permission.
However, for National Parks, Areas of Outstanding Natural Beauty, conservation areas and listed buildings, restrictions may apply and you’d need to check with the relevant body. In these cases it can be easier if panels will not be visible from the road (e.g. if the south-facing roof is to your garden).
If the panels are not flush with a roof you would also need to check and may need to get planning permission. For example if you’re mounting panels on a frame placed on a flat roof, or on the ground.
Yes, this is possible if you have a hot water tank. The electricity produced by a PV array can be diverted to an electric immersion heater. This needs to be controlled by a diverter unit that only turns the heater on when the PV array is producing enough output (so that it doesn’t use any mains electricity instead)
How much money or carbon this saves will depend on how you would otherwise heat water, and the cost of the equipment.
If your household or building has a large demand for hot water, then solar water heating may be a better choice, because you get more hot water from a smaller area of panel.
Carbon Savings
The carbon savings due to diverting PV to heat water, instead of using a gas boiler, have changed as the UK grid has decarbonised.
Several years ago, electricity use resulted in higher carbon emissions per unit than mains gas. Therefore, letting surplus electricity flow out to the grid, where it reduced electricity use in neighbouring houses, led to a greater carbon reduction overall than diverting it to heat water instead of using a gas boiler.
However, the large amount of wind power we have now, plus some solar and hydro power, means that UK electricity now has lower emissions per unit than mains gas. Therefore diverting solar PV to heat water instead of using gas will lead to a better overall carbon reduction than exporting to the grid.
However, using a heat pump to heat water uses less electricity overall, and so you could argue that if you move to a heat pump it’s better to use that to heat water! Then the PV electricity can be left to either be used by the heat pump – if the water heating cycle (maybe one hour per day) happens to be at a sunny time of day – or exported to the grid if not needed.
Financial Savings
Diverting the solar PV output to heat water in your home could save you some money. The payback time would depend on the cost of the diverter compared to the annual savings possible.
For a family house, hot water heating might use about 4kWh per day. If about 4kWh per day could be diverted from solar panels for about two-thirds of the year (when it’s sunny enough), that would be about 1000 kWh per year.
Back when gas cost about 4p per kWh then that saving would be about £40. If the diverter cost about £400 then the payback time is roughly ten years. A cheaper diverter would pay back more quickly.
However, gas is currently much more expensive – at over 10p per kWh. Therefore savings can be more like £100 per year and payback is much quicker.
If you only have electricity available to heat your water, then the saving will depend on the price per kWh you pay. That could bevery high on a normal day rate, but less on Economy 7 or other off-peak tariffs.
Solar Water Heating
Using a solar water heating system, you’ll need about 1 square metre (1m²) of panel per person to meet the hot water demand in summer, so maybe 3 to 4m² for a family house. Using PV panels you would need about 3 or 4 times as much roof area to get the same energy output. It would take perhaps half of the daily summer output of a 3.5kW (25m²) PV system to heat a cylinder of water.
Having both PV and solar water heating would make the best use of available roof area. Ideally, we would be integrating these technologies into new-build homes wherever they are appropriate – to maximise the contribution of solar energy and reduce carbon emissions.
A renewable energy technology could be ideal for pumping water where there is no mains electricity available, as a grid connection may be expensive and a diesel generator noisy and polluting.
Sizing a pump
The two main factors to consider when seeking a suitable pump are the flow rate – the amount of water that the pump will deliver, and the head – the height through which it will raise the water. These are related, as increasing the head will decrease the delivered flow. It’s important to minimise bends and other friction losses in pipework, as navigating these will require greater pressure, and as pressure and head are directly related, this effectively means a greater head.
Manufacturers’ technical data sheets will give the performance range of each pump, with graphs showing optimum combinations of flow and head. A pump sized properly to your needs will operate most efficiently. Suction pumps are limited to a depth of a few metres, so to draw water from a well or borehole, you’ll almost certainly need to lower in a submersible pump. Pumping wastewater or sewage necessitates one designed to handle drainage or effluent.
Small electric pumps for circulating water could cost tens of pounds, whilst those for drawing water from a well or borehole supply are likely to be a few hundred pounds. The main cost will be providing power to the pump, particularly when off-grid. Therefore, do first take all appropriate water-saving measures (such as spray-head fittings, mulches on plants to minimise water loss, etc) as these easily pay for themselves in the energy saved by reduced demand.
Off-grid electric pumps
Meeting a year-round water demand with a renewably-powered pump may require a combination of PV panels and a wind turbine, as this will balance energy production over the year. Sunshine and wind are naturally intermittent, so you may need some form of storage. Pumping water up to a tank (with demand then fed by gravity) during sunny or windy periods is more efficient than transferring the energy to batteries. If storing lots of water, you’ll need to balance the costs of a large tank (and supporting structure) against the costs of batteries (and their environmental impact and toxicity). An inexpensive control system can pump when needed, and otherwise divert power to batteries, giving extra backup facility.
The price of a small-scale renewable energy system will depend on the power and the maximum capacity needed. A very rough estimate is around £5 to £10 per installed watt. Siting generating equipment close to the pump minimises the cost and power loss incurred by cabling. As small turbines and PV panels usually produce power at 12 or 24 volts, a low-voltage pump would enable you to do without a costly inverter (for stepping up to 240 volts).
Mechanical pumps
For larger-scale pumping applications, you can avoid the losses in electrical systems by using mechanical power directly. See for example the question on our wind power page about wind pumps, or the question on our hydro power page about hydraulic ram pumps.
Signing up for a ‘green tariff’ from a company focused only on renewable energy is a great way to support the renewable energy industry. Changing your supplier is now very easy, and in most cases won’t make any difference to your supply.
One issue is that the small companies that specialise in renewable energy may not be part of the ‘Warm Home Discount’ scheme (although if they get enough customers they will be brought into it). This scheme gives a rebate to people at risk of fuel poverty, such as those receiving Pension Credit Guarantee Credit and some others. If this applies to you then you’ll need to stay with a larger provider to get this rebate.
Which green tariff?
We recommend choosing a company that only supports renewable energy. This means your money will not indirectly go to operate or build fossil-fuel power stations.
All electricity providers are required by the government to include some electricity from renewable sources. If they just offer a green tariff as one of a range of tariffs, then they may be simply charging a premium for electricity they’re legally required to produce! This is why we recommend companies that invest your electricity bill payments only in more renewable electricity.
If enough people sign up for renewable energy tariffs with these suppliers, then demand for renewable electricity will rise above the minimum government requirement. Therefore, as well as signing up yourself, encourage others to do the same.
The Ethical Consumer website gives a ranking based on the ethical and environmental record of electricity & gas suppliers. You have to be an Ethical Consumer subscriber to see the whole report, which gives more details.
I’m on a green tariff – so can I use as much electricity as I like?
It’s important to bear in mind that signing up to such a tariff does not mean you can leave all your lights on because it’s all zero carbon! If you use more electricity through your green tariff it means that less renewable electricity is left for those that are not on green tariffs. This means that more fossil fuel will be burned to meet their share of energy use.
Also, every means of generating electricity has some environmental impact, including the energy and materials that go into manufacture and installation. Energy saving measures are vital, because it’s them much easier to meet our electricity needs with energy sources such as wind farms, and wave & tidal power. Our Zero Carbon Britain project has a lot more details about how we can meet all our energy needs using only renewable energy.
It sounds great in principle to heat your house using a heat pump, and get the electricity needed using solar photovoltaic (PV) panels.
However, the UK climate makes this impractical. Very little solar energy is available at the time of the year when your heat demand is greatest. A fairly large 4kW solar PV roof (around 30m2) will produce around 15kWh of electricity per day in May or June, but only 3 or 4 kWh on a typical day in December or January. A heat pump may need about twice as much electricity as this, plus you’ll have several other electricity demands to meet.
A solar PV array can still be a good investment in itself, generating low carbon electricity to use in the home or to export and contribute to decarbonising the grid.
If you live in a rural area, you might have wind or hydro power available to you, which give more energy in winter. However, most homes don’t have a suitable site for these energy sources.
In a zero carbon future we will be able to run heat pumps using electricity supplied through the grid from renewable energy sources that generate power in winter. These are mostly large-scale – such as offshore wind farms and wave & tidal power.
We’re not able to recommend a particular make or brand of PV panel, as we don’t have the facilities to test and evaluate the thousands of panels that are available. However, see below for some information on the standard tests that are in place.
The tests are concerned with the quality and performance of the modules, not with ethical aspects such as environmental standards and workers’ rights. These can vary depending on where the module was manufactured, and note that modules made in the UK may actually just be assembled from components produced in other countries. Ethical considerations will also involve the other activities and financial investments of a company. For ratings of the ethical and environmental background of some of the main PV manufacturers, see the Ethical Consumer website.
PV panels should have certification to show they comply with the technical standards set out in the ‘BS EN 61215’ standard. This involves tests designed to assess the likely long-term strength and durability of a panel, and how it copes with different weather conditions, temperature changes, and so on. The Microgeneration Certification Scheme (MCS) has an accreditation process to ensure that these tests have been carried out by an independent laboratory. There are also audits to check that ongoing production is to the same standard as for the tested panels.
Maximum Power Point Tracking (MPPT) basically ensures the best output from PV panels in the available sunlight. You can have MPPT for a whole array, or for a set of panels (a string) or for each individual panel.
Note that if you have an unshaded roof and all the panels will be facing in one direction, then having individual MPPT devices is not usually worthwhile. They will not really affect output and will add extra complexity and cost to a system.
Some roof arrays are more complicated, with panels facing in different directions on different bits of roof, or with parts of the roof being shaded at sunny times of the day or year. In these cases, the use of MPPT should enable a better overall output. Whether the increased output covers the higher cost of installation will need to be weighed up in each case – looking at the benefits it will give versus any extra cost.
It’s definitely a good idea to have at least two different MPPTs if there are two different roof directions. This can be done using two different inverters, or one inverter using two different MPPTs. With a roof that is shaded at certain times it becomes more complex and would need to be evaluated.
Also, having extra pieces of equipment clipped to each individual PV module means that if they fail and need replacement, someone will need to access the roof. If each individual unit has only a 5% chance of failing in 20 years, then across a whole array of PV panels you could have a 50% chance of at least one failure in that time. Therefore you need to be getting a good additional output from using MPPT in order to justify the slight risk of failure and the cost of fixing it.
If a grid connection is already in place or available close by, then it makes more sense to generate renewable energy and buffer it with the grid. There’s rarely a benefit in going off grid when you don’t need to.
However, in place where there’s no grid connection or it would be very expensive to connect, a small wind turbine and some photovoltaic (PV) solar panels can be a good alternative to a diesel generator (which will be noisy and polluting and have ongoing fuel costs).
If you’re off-grid and need power all year round, wind and solar complement each other well as there’s more wind in winter and more sun in summer. If you only need power in summer, you could get awaty with only using solar power.
Considerations for siting a wind turbine or solar photovoltaic panels are the same as with grid-connected systems, so see our pages on these. You need an unshaded and roughly south-facing site for solar, and somewhere with strong, consistent wind speeds for a turbine.
Sizing an off-grid system
If living off-grid you’ll need to store wind & solar powered electricity in batteries. To have sufficient electricity for your needs, you must first estimate how much electricity you’re likely to be using. You can do this by estimating the total energy consumption of all the electrical appliances you intend to run.
The power rating in watts (marked on the back of the appliance) multiplied by the time (in hours) that it will be used each day, gives the energy (in watt-hours) that you will need per day in order to run that appliance. Add all these figures up to see what size system you require.
The more energy you need, the bigger and more expensive the system becomes. Therefore, make sure you are using energy efficiently, and consider whether or not all the appliances are necessary. Fitting low-energy light bulbs and using other fuels for heating (perhaps wood fuel) and cooking will make a big difference to the overall cost.
Batteries
Batteries are essential in most off-grid wind or solar systems, but are expensive and will deteriorate. They store low voltage (up to 48V) DC electricity and need to be protected from over and under charging with a suitable controller. Lead-acid batteries were common in off-grid projects, but now lithium-ion are becoming more common. The latter have a longer lifetime but are more expensive – so lifetime costs may be similar.
Deep cycle batteries are preferable for renewable energy systems, as they are designed to have up to 80% of their charge repeatedly removed and replaced over a period of 5 to 15 years (or 1000 – 2000 times). Vehicle batteries are not suitable as they are designed to give a short burst of high current and be recharged immediately. They will perform poorly if allowed to discharge more deeply. Do make sure you follow proper safety procedures when dealing with batteries.
Inverters & Cables
To run some appliances you’ll need an inverter to convert from 12 volts DC to 230 volts AC. There are different types of inverters for grid-connected and off-grid systems. They can be expensive, and rise in cost as the maximum power that they need to be able to cope with increases, so it could be worth trying to run DC appliances where possible (for example, low-voltage lighting).
Solar PV panels and small wind turbines usually operate at low voltages (e.g. 12 or 24 volts). The voltage drop in wires can have a significant effect at these levels. Cables must be thick enough to minimise this drop and carry the required current. As cables are expensive, this can affect wind turbine siting.
Related Pages
Related events
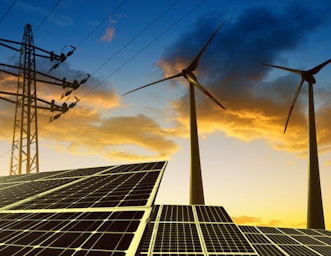
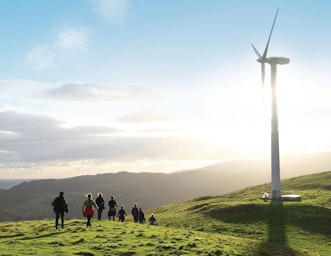
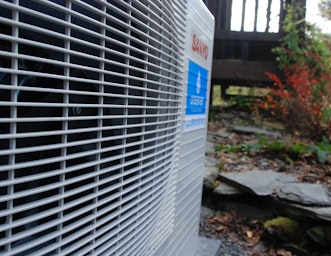
Renewables for Households: Heat Pumps (Only two places left)
8th March 2025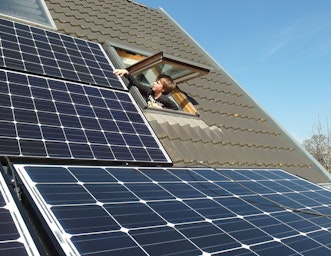
Renewables for Households: Solar PV
12th April 2025Related news
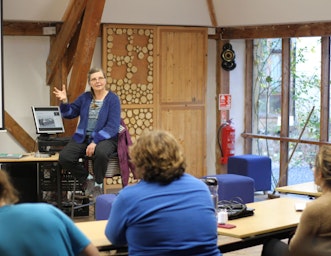
CAT Conversations – Dr Frances Hill
10th July 2024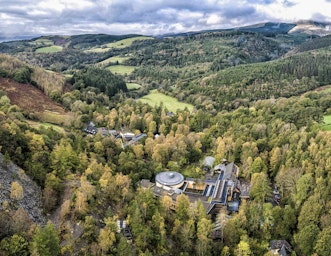
CAT Stories – Aber Food Surplus
23rd May 2024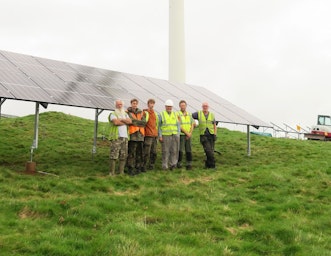
Taking back power
9th April 2024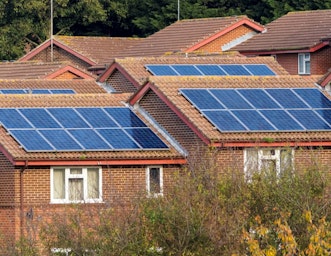
Why we teach… community solar
9th April 2024Study at CAT: Related Postgraduate Courses
Related Books
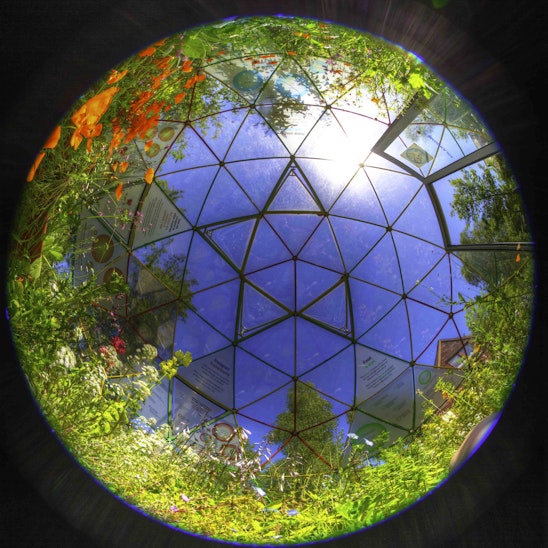
Did you know we are a Charity?
If you have found our Free Information Service useful, why not read more about ways you can support CAT, or make a donation.
Email Sign Up
Keep up to date with all the latest activities, events and online resources by signing up to our emails and following us on social media. And if you'd like to get involved and support our work, we'd love to welcome you as a CAT member.